Storage temperature
Long-term storage of Biological sample usually uses the lowest possible temperature to reduce biochemical reactions within the sample and improve the stability of various components in the sample.
Common storage temperatures for biological macromolecules, cells, tissues and organs are -80°C (ultra-low temperature refrigerator), -140°C (liquid nitrogen gas phase or deep-freezing refrigerator) and -196°C (liquid nitrogen liquid phase). The lower the temperature, the better the sample. The longer the stable storage time. 0~-60°C is the crystallization temperature of water, which can easily cause damage to the microstructure of cells and tissues. This temperature is generally not used to preserve tissues and cells. Some purified biological macromolecules can be stored stably for a certain period of time at 0 to -60°C. However, biological macromolecules in tissue samples are affected by various factors in the cell tissue, and the stability may be significantly reduced, so they are usually not used in sample libraries. 0~-60℃ is used as storage temperature.
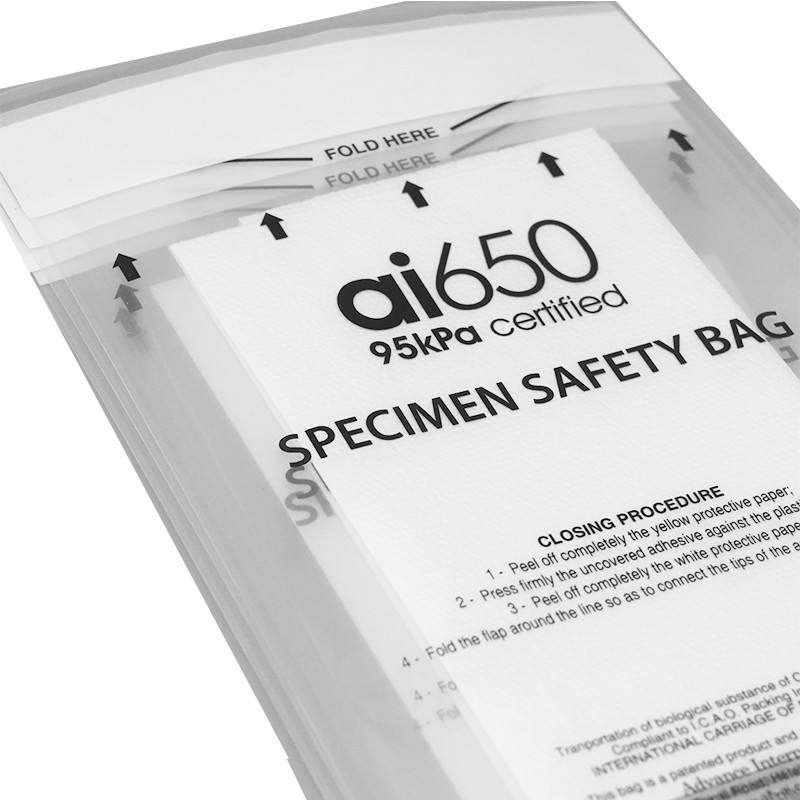
-80℃ Biological sample storage
-80°C is lower than the crystallization temperature range of water, which is more harmful, and is also the temperature that commonly used ultra-low temperature refrigerators can reach. Based on factors such as ease of operation, storage capacity, and cost, this temperature is also the temperature that most biological samples currently store. Common temperatures at which molecules become active. However, it is still unclear how long the activity of different biological macromolecules can be maintained at this temperature. The stability of DNA in tissues can be maintained at -80°C for several years or longer. But for RNA, it is easy to be gradually degraded by RNases that are widely distributed in cells and various tissues. In different cells and tissues, the length of time that RNA can be stably stored varies greatly, but it generally does not exceed 5 years. , in some sensitive experiments, RNA has undergone measured degradation in less than a year at -80°C. Therefore, in order to preserve RNA activity for a long time, it is recommended to use a lower temperature, or to use a small part of the sample to extract RNA and the remaining sample. Synchronized storage. Biological macromolecules such as proteins and lipids in other samples can also be stored at -80°C in the sample, but the time varies and the stability gradually decays. If it is necessary to protect a specific biological macromolecule known to be present in the sample, a stabilizer for that molecule can also be added. If the biological macromolecules to be preserved in the sample are not determined, it is recommended to store them at a lower temperature. In addition, currently common large-scale automated sample storage and withdrawal equipment can only be used with -80°C ultra-low temperature equipment, and cannot yet be used with liquid nitrogen equipment. For example, a part of the sample copies (~9.5 million) are stored at -80°C as working samples and stored using automated equipment; the other part of the copies (~5.5 million) are stored in another place in the liquid nitrogen gas phase for safety backup, and the samples are stored manually. Pick.
-140℃ Biological sample storage
-140°C is lower than the glass transition temperature of water (~-136°C), and is also the temperature that liquid nitrogen gas phase and deep-freezing refrigerators can reach. The biological activity of samples is greatly reduced in this temperature range, which is the best time to store samples. Ideal temperature for cell activity. Similar to the phase change temperature of an ice-water mixture that can be maintained at 0°C, the liquid and gas phase nitrogen in an insulated liquid nitrogen container should be maintained at a phase change temperature of -196°C. But in fact, because the lid of the liquid nitrogen container is not tight enough, a temperature gradient is formed between the liquid nitrogen level and the mouth of the liquid nitrogen container. The National Cancer Institute recommends that the temperature at the mouth of the liquid nitrogen container should be kept below -140°C, and samples with undetermined future uses should be stored in liquid nitrogen gas phase mode to protect cell activity in the tissue.
The deep-freezing refrigerator is electrically refrigerated and does not require liquid nitrogen. The stable temperature after being filled with samples is usually below -140. Compared with the use of liquid nitrogen gas phase, its advantage is that it does not require frequent addition of liquid nitrogen and is easy to maintain. However, the cooling rate of electric refrigeration is lower than that of liquid nitrogen. Once the container is opened to pick up and place samples, it is easy to cause a wide range of temperature fluctuations, and the temperature recovery time is relatively long, so it is more suitable for situations where samples are rarely opened and placed. In addition, electric refrigeration refrigerators must ensure power supply. Liquid nitrogen equipment is recommended to provide backup storage in the event of a power outage.
-196℃ sample storage
-196°C is the temperature at which liquid nitrogen evaporates, so only liquid nitrogen liquid phase preservation technology can reach this temperature. The life activities in the sample basically stop at this temperature, and the stability of the sample can be preserved for a long time. It is the most effective method for long-term preservation of cell activity, complex structure and activity of tissues and organs in samples, and is widely recognized. Compared with other different temperature freezing modes, liquid-phase storage of samples in liquid nitrogen containers requires further protection from cross-contamination between samples. The National Cancer Institute recommends the use of spiral cryovials for packaging samples. However, during the cooling process, the sample is suddenly cooled when transferred from the ultra-low temperature refrigerator (-80°C) to liquid nitrogen, causing inconsistent shrinkage of the cryopreservation tube cap and tube body, which can easily cause liquid nitrogen to penetrate into the cryovial tube, thereby increasing the risk of cross-contamination between samples. risk. One of the solutions is to use a special cryopreservation tube sleeve to heat shrink seal each cryopreservation tube, or use a sealing film to wrap 2-3 times at the interface between the cryopreservation tube cap and the tube body before storing. The former method will lengthen the tube body and require a taller freezing box. The second method will make the tube body slightly thicker. It is recommended to use a conventional freezing box with a size of 10×10 at the bottom.

Programmed freezing/stepwise freezing
Ice crystal damage and solution damage can be minimized by controlling the cooling rate of the sample. The cooling speed can be controlled by stepwise freezing or programmed freezing at different temperatures. Compared with step cooling, the programmable cooling device can more accurately control the cooling rate of the sample itself by constantly adjusting the flow rate of liquid nitrogen sprayed into the freezing storage space. For example, when a liquid sample transforms into a solid at 0-5°C and undergoes a phase change, heat will be generated, causing the sample to warm up and causing additional damage to the sample. The programmable cooling device can increase the cooling intensity during phase change to avoid this damage. Programmed cooling instruments are widely used to freeze sperm, eggs, fertilized eggs, stem cells, skin and other samples. About 300,000 to 400,000 IVF babies around the world have used this method. A cryopreservation program usually includes several stages such as slow freezing and quick freezing. In the presence of a cryoprotectant, the common freezing rates are slow freezing 0.3-1.5°C/minute and quick freezing 5-8°C/minute. Many programs use slow freezing in the range of 0 to -40°C. However, the specific cooling procedures may vary greatly depending on the frozen samples.
Vitrification
The theory of vitrification was first proposed by Luyet. The solidification of liquid can be divided into two forms: one is crystallization, in which the molecules in the solution are arranged in an orderly manner; the other is amorphous, that is, vitrification, in which the molecules in the liquid are in a disordered state and remain in the state before solidification. . During normal freezing, water easily forms crystals inside the cells, causing crystal damage, and forms crystals outside the cells, causing solution damage. However, under the conditions of ultra-rapid vitrification, both the inside and outside of the cells are vitrified and solidified. No ice crystals are formed or only very small ice crystals are formed, which will not cause damage to the cell membrane and organelles, and the cells will not be exposed to high concentrations of solutes for a long time. damaged by exposure. However, the ultra-high-speed cooling required for vitrification is difficult to achieve under conventional experimental conditions. In 1981, Fahy proposed the method of using high-concentration cryoprotective solution (vitrification solution) to greatly reduce the requirement for cooling rate. In 1985, Rail and Fahy completed the vitrification cryopreservation of mouse embryos, achieving a breakthrough in the use of this technology. .
Vitrification is a newer technology that is suitable for samples that are difficult to process by conventional procedures, such as complex tissues and organs. However, this technology has not yet shown particular advantages for samples that can be better processed by conventional program cooling (such as cells and very small tissues). Normal vitrification of water requires extremely fast speed, which is difficult to achieve in conventional laboratories. In order to reduce the vitrification cooling requirements of the tissue, a high concentration of cryoprotectant needs to be added to the sample. The commonly used concentration is about 40% to 60% (W/V). Even a few minutes can cause significant damage to cells and tissues. Therefore, although vitrification reduces ice crystal damage and solution damage during the freezing process, it did not show better results in some comparative experiments. On the contrary, its complicated operation caused inconvenience. Currently, in vitrification, the main method to reduce cryoprotectant damage is to use mixtures of different cryoprotectants and ice blockers. Through this improvement, Twenty-First Century Medicine successfully transplanted frozen and thawed rabbit kidneys into rabbits and maintained normal function.
Snap freezing
Flash freezing is often used to protect purified biomacromolecules or tissue samples for future extraction of biomacromolecules. The method is to put the sample directly into liquid nitrogen, process it for a short time, and then transfer it to an ultra-low temperature refrigerator or a lower temperature environment for storage. A common example is freezing tissue for future nucleic acid extraction. This approach will damage most cells and fine tissue structures and cannot be used to preserve the viability of cells and tissues within the sample.
cryoprotectant
Cryoprotectants refer to substances that can protect the microstructure of cells and tissues from freezing damage. They are usually formulated into solutions of a certain concentration. Adding cryoprotectants to cell suspensions can protect cells from solution damage and ice crystal damage. The cryoprotectant combines with the water molecules in the solution to cause hydration, weakening the crystallization process of water, increasing the viscosity of the solution and reducing the formation of ice crystals. At the same time, the cryoprotectant can maintain a certain molar concentration inside and outside the cell, reducing the The concentration of electrolytes in an unfrozen solution protects cells from solute damage. Usually only red blood cells, most microorganisms and very few nucleated mammalian cells are suspended in water or simple saline solution without cryoprotectant and frozen at the optimal freezing rate to obtain viable cryopreserves. However, for most nucleated mammalian cells, there is no optimal freezing rate without the addition of cryoprotectant, and viable frozen products cannot be obtained. For example, if mouse bone marrow cells are suspended in a balanced salt solution without cryoprotectant and frozen at a freezing rate of 0.3 to 600°C/min, more than 98% of the cells will die; while adding glycerol cryoprotectant for cryopreservation At this time, more than 98% of cells can survive.
Cryoprotectants can be divided into permeable and non-permeable categories based on whether they penetrate the cell membrane. Osmotic cryoprotectants are mostly small molecule substances that can penetrate into cells through the cell membrane. This type of protective agent mainly includes dimethyl sulfoxide (DMSO), glycerol, ethylene glycol, propylene glycol, acetamide, methanol, etc. Its protective mechanism is to penetrate into the cells before the cell freezing suspension is completely solidified, generate a certain molar concentration inside and outside the cells, and reduce the concentration of electrolytes in the unfrozen solution inside and outside the cells, thus protecting cells from damage by high-concentration electrolytes. , the water in the cells will not leak out excessively, avoiding excessive dehydration and shrinkage of the cells. Currently, DMSO, glycerin, ethylene glycol and propylene glycol are commonly used. DMSO penetrates cells quickly and has a better protective effect during cryopreservation. It is commonly used. The commonly used concentration is 5%-10%. However, DMSO itself has obvious damage to cells, especially at a temperature of 4°C. Therefore, it is not recommended to add DMSO to the sample and leave it at this temperature for a long time. It is usually used in conjunction with a programmable cooling device or a gradient cooling box to achieve continuous and uniform temperature. reduce.
Non-permeable cryoprotectants cannot penetrate into cells and are generally macromolecular substances, mainly including polyvinylpyrrolidone (PVP), sucrose, polyethylene glycol, dextran, albumin and hydroxyethyl starch. There are many hypotheses for its protective mechanism. One possibility is that macromolecular substances such as polyvinylpyrrolidone can preferentially combine with water molecules in the solution, reducing the content of free water in the solution, lowering the freezing point, and reducing the formation of ice crystals; at the same time, due to its The large molecular weight reduces the electrolyte concentration in the solution, thereby reducing solute damage.
Different cryoprotectants have different advantages and disadvantages. The current trend is to use combinations of more than two cryoprotectants. Since many cryoprotectants protect cells at low temperatures, they are harmful to cells at room temperature. Therefore, the cryoprotectant should be removed promptly after the cells are rewarmed.
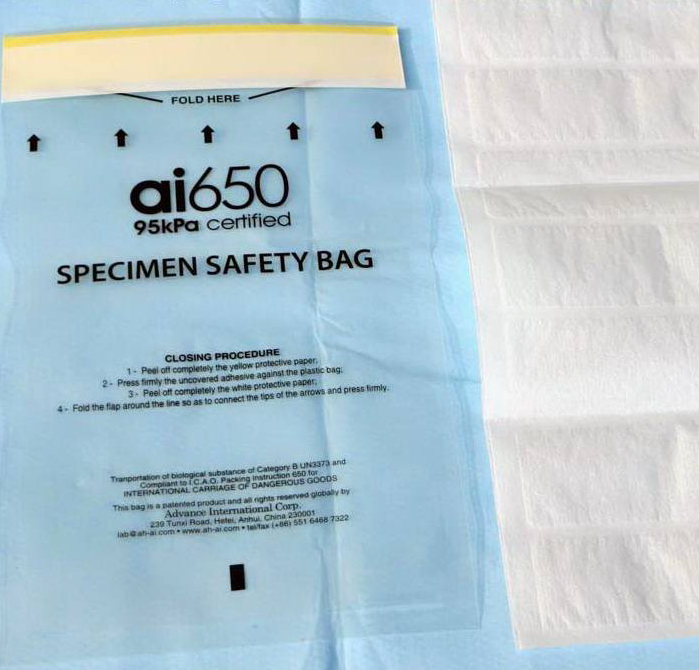